Draper Laboratory Solving Military Energy Challenges
While previous Executive Orders and Federal legislation have been useful in moving Federal Agencies toward sustainability and, in many cases, have spelled out numerical targets to be achieved, the 2010 National Defense Authorization Act requires the Department of Defense (DoD) to use renewable sources for 25 percent of its facilities by 2025. DoD itself highlighted that “reducing the demand for energy must be the most immediate operational energy priority for the Department” in its Operational Energy Strategy released May 2011.
In meeting these requirements, DoD has identified a preliminary challenge to accomplishing these objectives: It currently lacks sufficient data and analysis of its operational energy use. In addition, it needs to better understand its energy consumption – the where, how and why of usage – in order to better manage consumption. In other words, it can’t improve what it doesn’t know.
Improving the Unknown
Military installations pose unique energy management and distribution challenges. For example, DoD test and training facilities typically include a housing or cantonment area; mission support facilities, such as mission control centers, laboratories, office space; and test and training ranges. The ranges often cover significant land area over very challenging terrain and support multiple simultaneous tests of the newest technologies being considered for fielding to America’s armed forces. White Sands Missile Range, for example, has ranges extending more than 3,200 square miles. In addition, hundreds of remote test sites are routinely used. Installations often operate on fixed budgets and have critically aging energy infrastructures. These remote operations typically use diesel generators, resulting in air quality issues as well as increased logistical complexity for refueling operations.
Further complicating the situation is the support of many different activities from multiple DoD and non-DoD agencies at a single range. Since no infrastructure exists to track energy usage by multiple range tenants, tracing energy usage to these agencies is difficult or impossible at some installations. And, due to the aging infrastructure and the large operational area of DoD’s test and training ranges, the information to make decisions about energy distribution, reimbursement and investment is often not available or not presented in a fashion suitable for rapid analysis and decision-making.
The complexities of military installations require novel data analytics and data fusion capabilities for effective energy management and decision-making. These efforts should be initially focused on collecting, analyzing and visualizing critical data on energy consumption by end-users. Technologies for such capabilities include: real-time sensing and monitoring, advanced metering, network communications, demand-side management systems and the necessary decision and control systems to shape the demand profile. A typical program requires a comprehensive site survey and data collection effort throughout at least a 12-month period to fully assess, analyze and document current energy systems and policies. This effort is then followed by detailed design development of a comprehensive networked energy monitoring and management system that is integrated with the site’s transmission and distribution systems.
Sophisticated software tools are needed to carry out the multitude and wide variety of tasks required for effective energy management. Draper Laboratory has recently adapted software previously developed to control autonomous and crew-assisted operations on the International Space Station (ISS) to do just that.
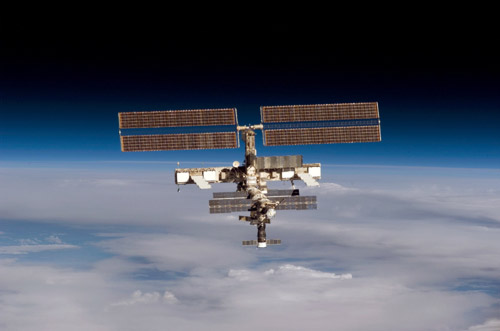
Photo Credit: NASA|Backdropped by the blackness of space and Earth’s horizon, the International Space Station moves away from Space Shuttle Discovery.
This User Interface Language software, known as Timeliner™, allows NASA to enable autonomous operations of a multitude of functions on ISS and is installed on the Space Station’s Command and Control and Payload Control Processors. It was first used in 2003 to autonomously activate and to control payloads in the microgravity payload experiment. It is also used to automate other ISS procedural tasks performed by human operators or those that execute a control process, which include vehicle control, performance or pre-flight and post-flight subsystem checkout, and handling of failure detection and recovery. Timeliner’s English-like development language, or MetaScript, has built-in interconnectivity to network devices, servers, databases and application software. It provides an ultra-reliable runtime platform for a limitless distributed execution environment that can process millions of simultaneous complex and disparate events in real time.
With the addition of recent advances in data analytics and data fusion, Timeliner™ is running today in commercial power plants, collecting data and controlling processes across the entire production spectrum. Equipment maintenance and in-use monitoring are critical for improving power plant reliability and efficiency. Automated measurement and advanced diagnostic and control systems have been deployed recently in coal-fired plants and natural gas-combined cycle plants in the U.S. and have demonstrated success in monitoring the thousands of critical parameters vital to achieving high reliability. Technology solutions like this enable replacement of the periodic, manual measurement and maintenance procedures typically used today with high value analytics and decision-making.
The same advances being used to improve power generation in the electric utility sector have broad applicability to the above-described issues facing DoD. Mainly, the military needs sophisticated, high-level approaches and corresponding infrastructure to collect data and to turn it into high-value information through data analytics. This data can be further enhanced when fused with other data, such as disparate pieces of information and, in turn, can be used for critical decisions and control actions as part of an overall hierarchical energy management system.
Reaching Integrated Net Zero
Data and high-value information of the type described above are critical for the military to achieve their sustainability mandates. Motivated by various Executive Orders and the 2010 National Defense Authorization Act, a significant number of military installations have stepped-up to exceed their explicitly mandated requirements. Some are gearing up to go even further and achieve “Net Zero” in the category of energy, waste or water. An even more select group of installations are working to achieve the ultimate in sustainability: “integrated Net Zero.” In such comprehensive efforts, a solid data and information foundation regarding energy and material flows is vitally important.
Achieving Net Zero in all three categories – energy, water and waste – poses challenges that are more than just simply the sum of the individual efforts. High level system architecture is required to manage complex energy and material flows due to their interdependencies. System models that capture these linkages enable intelligent control algorithms to maximize the benefits of an integrated approach to Net Zero. In addition, opportunities exist where a single technology can be deployed toward achieving Net Zero in more than one category. The direct carbon fuel cell is an example, which can consume waste materials that contain carbon while simultaneously producing electricity for use on-site, i.e., waste-to-electricity.
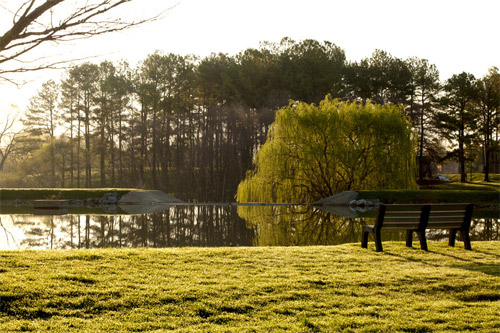
Photo Credit: U.S. Army|Nallin Farm in Fort Detrick which is the recent selection to be an Army pilot installation for Net Zero Energy and Waste initiatives.
A Direct Carbon Fuel Cell (DCFC) is a type of fuel cell that electrochemically combines solid carbon and oxygen to produce carbon dioxide (CO2) and electrical power. Theoretically, a DCFC offers major advantages when compared to other types of fuel cells as well as combustion-based power plants. Reasons include: reduction in number of moving parts needed for the system; emission of a pure CO2 exhaust ready for sequestration; generation of controllable power output to meet electrical demand; and, above all, higher thermal efficiency.
Existing coal power plants achieve efficiencies of approximately 30 percent whereas a DCFC system could exceed 60 percent, thus effectively doubling the power output at just one-half the CO2 emissions for the same quantity of fuel (without sequestration). Scientists from Lawrence Livermore and Brookhaven National Laboratories have calculated higher heating value thermal efficiencies from DCFCs of 80 percent for petroleum coke, 67 to 75 percent for natural gas, 72 percent for heavy fuel oil and 68 percent for lignite coal. These high thermal efficiencies, coupled with lower fuel costs, represent a significant cost savings potential. For example, coal and petroleum coke (a byproduct of oil refining) today sell in the range of $2.50 to $3 per million BTU compared to approximately $5 per million BTU for natural gas (Electric Power Monthly – U.S. Energy Information Administration), which is the predominant fossil fuel choice for new generation capacity additions.
In addition, a DCFC is fuel flexible, meaning that the carbon fuel source can be derived from a multitude of sources including coal, biomass, diesel fuel, JP8 and organic waste, such as plastics. For example, Direct Carbon Technologies, LLC, of Palo Alto, Calif., has demonstrated DCFC operation on several eastern bituminous coals as well as activated carbon and biomass (almond shells). CellTech Power, LLC, out of Westborough, Mass., has developed kW size prototypes for portable power and military tactical power, which operate directly on various hydrocarbon fuels, including natural gas, JP8 and waste plastic. CellTech Power prototypes have successfully operated for extended time periods in excess of 2000 hours.
Generating Power Reliability & Efficiency
Forms of DCFCs have been in existence since 1894. However, practical designs that can operate continuously for an extended duration are still under development. Typical issues include: electrode degradation (corrosion), electrode clogging, introduction of fuel into the system, low power density, generation of ash that needs to be removed and electrolyte poisoning. Each is a major concern with a limited solution space. In conjunction with a university collaborator, Draper is actively developing a hydrodynamic direct carbon fuel cell, which offers potential solutions for these vexing issues. The near term goal is to demonstrate a multi-cell stack at a current density of 100 mA/cm2 with 60 percent fuel cell efficiency that is capable of operating in excess of 1000 hours.
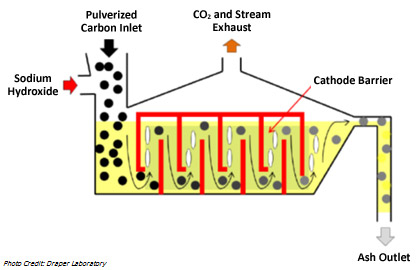
.
This work to date has demonstrated feasibility of the hydrodynamic DCFC concept. The approach uses gas lift, in this case steam, to fluidize the electrolyte/fuel mixture preventing ash buildup from blocking the anode electrode. Steam is preferred since it suppresses the formation of carbonates, is readily available and is easy to separate from CO2. The anode flow field is designed to accommodate a slurry flow while minimizing potential for clogging. To affect a compact, affordable yet robust design, the anode flow field is as thin as possible. The notional concept to the right shows the gas lift technique (akin to a jet pump), which facilitates slurry movement in the anode flow field.
DCFC technology could benefit military and civilian applications. Three primary areas include:
- large-scale commercial and behind-the-fence power generation,
- remote fuel-flexible portable power generators and
- organic waste disposal and waste-to-energy.
Larger military installations already generate power on-site or are candidates for adding power as they move to various strategies for achieving Net Zero. For example, Fort Bliss has a 90 MW peak power requirement and is planning to add various forms of behind-the-fence power generation, including renewable and waste-to-energy. The payoff for any large-scale power generation is driven by the high efficiency of DCFC systems. In 2010, coal fired power plants were used to generate 45 percent of electricity in the United States, which is approximately $100 billion in revenue. These plants typically operate between 30-35 percent Higher Heating Value fuel efficiency from coal. DCFC systems, as mentioned previously, could operate in excess of 60 percent, which translates to potentially a factor of two reduction in fuel cost. In the future, a significant monetary value will exist for the associated CO2 emission reduction, which today is estimated to be in the range of $50 to $100 / ton of CO2. In 2010, coal fired power plants in the United States emitted approximately 1.9 billion tons of CO2, which translates to a value north of $50 billion.
Remote, fuel-flexible power generation is another application area for DCFC technology. Unlike many combustion systems, a DCFC can be fueled with any carbon—rich fuel source including wood, grass, tires, plastics and even animal waste. This is critical for power generation in remote (or mobile) locations where no electrical transmission lines exist and where access and/or oil transportation is expensive or dangerous. A specific example is a U.S. military Forward Operating Base (FOB), a secured forward military position used to support tactical operations and to enable improved reaction time.
As it exists today, the U.S. military is an expeditionary force that depends heavily on FOBs being deployed quickly and effectively anywhere across the globe. These bases consume large amounts of electrical power fed by on-site diesel generators. A typical base containing 1100 people will have a peek power requirement of approximately 4,000 kW and a 24-hour consumption of more than 50,000 kWh.
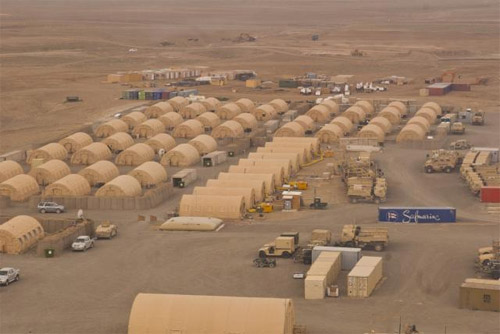
Photo Credit: Karen Parrish, DoD|Forward Operating Base Sharana, Afghanistan, is headquarters for Task Force Currahee’s 4th Brigade Combat Team, 101st Airborne Division. The brigade is responsible for security operations in Paktika province.
This size base would contain one large 750 kW diesel-powered generator coupled with several secondary generators ranging from 30 kW up to 250 kW. Combined, these generators can consume almost 5,000 gallons of fuel per day. Over a month, a 2,500-gallon military fuel tanker (HEMTT) is required to make 59 fully loaded deliveries or two deliveries per day.
Direct Carbon Fuel Cell technology may offer a unique solution to this problem. Supplementing existing diesel power generation with DCFC systems could reduce fuel costs but, more importantly, also allow the base to generate power independently by using local resources. Fuel sources could include: wood, biological waste, and organic garbage like plastics and paper products. This would reduce the frequency and dependence on fuel convoys.
A third DCFC application is waste disposal. Military installations generate large quantities of biological waste, which is costly to dispose. An onsite DCFC could consume these waste products while also supplying electrical power for base needs. This “double benefit” solution is highly desirable compared to current alternatives.
Leading the Way
The U.S. military can meet the objectives set by DoD and legislative directives to ensure a sustainable force for the future. Capturing data on its current operational energy use is the crucial first step to begin analyzing how it can reduce energy consumption, integrate renewables and deploy current technology solutions to realize a Net Zero operation while meeting the needs of DoD and addressing the complexities of military installations. The reductions to strategic, operational and tactical costs across the board will be significant. The military can lead the way. Our country depends on it!